Introduction
In food plants, antiseptics like solution of hypochlorite and quaternary ammonium molecules have been utilized to eliminate contaminating bacteria, especially those that cause foodborne illnesses. Because of possible health risks, several sanitizers (e.g., formaldehyde) have been restricted or prohibited. However, due to rising disease epidemics and the advent of novel food-borne pathogens, the demand for powerful antimicrobial medicines has grown in recent times (Ogunsona et al., 2020). Infections done by Escherichia coli O157:H7 in refrigerated minced beef and burger, hepatitis E virus (HEV) and Listeria monocytogenes in sausages and in freezing strawberry have reignited attention in efficient control techniques. As a result, the food industry is looking for sanitizers that are efficient against prevalent and new diseases while also being functional and safe in a number of food manufacturing operations. Ozone (O3), for example, has been used as a disinfectant in several European-water systems since the turn of the era (Gottschalk et al., 2009). The use of ozone as a powerful agent of oxidation in foodstuff and other sectors has several benefits. It has the ability to reduce microbial loads, hazardous organic compound levels, chemical-oxygen-demand and biological-oxygen-demand in the atmosphere. Certain non-biodegradable organic compounds found in wastewater are converted to biodegradable ones by ozone (van Leeuwen et al., 2009). Because the molecule immediately disintegrates to oxygen, employing ozone reduces the deposition of inorganic waste. Ozone is a suitable decontaminator for guaranteeing the bacteriological hygiene and safety of food items due to its strong oxidizing potential and spontaneous disintegration (Perry et al., 2019). Food and food components including’s milk, animal products, albumin, gelatin, and casein had been investigated for the conservation of ozone up to the decade’s beginning. Ozone was used for purifying and artificial ageing of alcoholic drinks such as wine and liquor, sanitation of brew and cider production facilities, odour control, and medicinal therapy. The majority of recognized applications, on the other hand, focused with the purification of drinking-water similarly municipal wastewater. The utilization of ozone within food sector in the U. S was previously limited. It was largely used to remove iron, manganese, colour, favours, and smells from water (Gottschalk et al., 2009). The US Food and Drug Administration declared ozone to be commonly regarded as safe to be used as a sanitizer in bottled-water in 1982, with certain limitations (Rice et al., 2002). Reconditioned water may be recycled in poultry fridges, according to the US Agriculture department. It should be disinfecting or sanitizing agent in food manufacturing in the U.S, according to the acceptance of the US FDA (Artes et al., 2005). The legislation of the Food-Safety-Modernization-Act in the U.S. may result in the formulation of recommendations for disinfectant treatments to combat foodborne microorganisms (USFDA, 2011). Academics and food processors have been interested in ozone uses as a result of these regulatory changes. As a result, a recent and detailed review paper will be useful in determining the application of ozone within present food sector. Ozone technology’s multi-processing capabilities make it efficient against numerous pathogens in vegetables, meat, and fruits while also conducting water treatment (Mohamet et al, 2021; Pandiselvam et al., 2022). Since ozone was given permission to be used in food processing within U.S, there has been a great deal of interest in researching the effectiveness and potential of ozone application in various food manufacturing plants. It has been examined for a variety of applications in numerous industries, such as the fruit and vegetable sector (Karaca & Velioglu, 2007). This article differs in that it discusses ozonation as a preservative in dairy, fruits and vegetables, poultry, cereals, and fresh produce in depth, while most published papers only discuss one of these areas.
Role of Ozone-Based Technology in Food Safety and Properties of Ozone
Ozone is a potent oxidant which has many foods uses to advance the safety of foodstuff. Improve quality, safety and extending the storage life of the food product is a significant issue. Treatment of ozone is considered an eco-friendly and cost-operative food processing method (Tiwari et al., 2010). Ozone has been utilized to handle a wide variety of agricultural products, like fresh produce (e.g., vegetables and fruit), dairy products, poultry, and food grain products (Cullen et al., 2009; Tiwari et al., 2010; Varga & Szigeti, 2016). Ozone is seen to be an efficient food preserving agent as it causes mycotoxin damage, microbial inactivation, and insect killing (Li et al., 2015). Ozone cannot be preserved for substantial amounts of time; thus, it should be created when necessary. Ozone may be created on purpose using a variety of approaches. This involves photochemical processes that use ultraviolet light but usually result in small amounts of ozone, water electrolysis to create hydrogen gas and ozone, such as corona discharge (Prabha et al., 2015). Among all corona discharge is a most effective method used and can create relatively high amounts of ozone. The main benefit of utilizing ozone for food production is that it leaves no residue on application due to accelerated decay. This aspect makes the production of ozone especially appealing as a more environment friendly method (Paes et al., 2017; Prabha et al., 2015). Ozone has quite a wide range of antimicrobial pursuits, due to its excellent reactivity, which is caused by free radicals’ oxidizing ability. Ozone decomposes into hydroperoxy, superoxide, and hydroxyl radicals because of its instability in both the gaseous as well as aqueous phases (Manousaridis et al., 2005). Bacterial inactivation by ozone action is a complex process that involves an ozone attack on the cellular membrane components (protein, unsaturated fatty, respiratory enzymes), cytoplasm, spore coat, and virus capsids (Guzel-Seydim et al., 2004). Ozonation is widely utilized for purpose of decontamination of drinking water. Ozone is a very powerful disinfectant, yet it doesn’t leave a chemical residue on food or surfaces in touch with food since it breaks down swiftly into benign compounds, lowering the company’s environmental impact and expenses (O’Donnell et al., 2012). Another benefit of using ozone in food processes is that it can be produced onsite on demand, unlike traditional chemical sanitizers, and it doesn’t need to be transported or stored (Cullen and Norton, 2012).
Antimicrobial Action of Ozone
The problem of food contamination necessitates ongoing food control at every stage of manufacturing (Brodowska et al., 2018). Ozone significantly and specifically oxidizes the cell-wall with in bacterium cytosolic membrane. Ozone is utilized to disinfect manufacturing areas, sterilize surfaces, and fumigate industrial gear. Ozone helps to eliminate microflora, including germs, extending the shelf-life of food (Islam et al., 2022). According to Smith and Pillai (2004), ozone has biocidal effects on bacteria like Zygosaccharomyces bailii. Ozone has been used in the processing of fruits and veggies to get rid of pathogenic bacteria, pesticides, mycotoxins, and chemical remnants (de Souza et al. 2018). Ozone was found to increase carrot storage life and reduce the fast rise in total-soluble-solid during storage in a research by de Souza et al. (2018). The pH of juices, ingredients (such as emulsifying agent and amount of sugar), composition, temperature, frequency of ozone dispersion, the concentration of organic substances, and the total-solids all affect how successfully ozonation inactivates bacteria in fruit juice. The chemical makeup of juice also has a role in how much O3 affects the value of fruit juice in addition to ozone concentration and exposure time (Choi et al., 2012). Stiffness is an important textural attribute of fruits and vegetables. The use of ozone spray to preserve strawberries and tomatoes was investigated by Jaramillo-Sánchez et al. (2019). In another study 21 dairy-isolated Pseudomonas spp. strains developed a biofilm that was inhibited and eliminated using gaseous-ozone (50 ppm over 6 h), according to Panebianco et al. (2022).
Pilot Scale Ozone Level Application
Controlling the disinfection procedure has been extremely difficult due to the various pollutants in recycled water resources and the prolonged feedback of microbiological detection. To assess and improve disinfection technologies and mechanisms, sensitive and online surrogates for signalling microbial inactivation effectiveness must be identified. The inactivation of microbiological markers during ozone disinfection is examined in this study during the course of a 5-month pilot trial. It has been determined that intracellular-adenosine-triphosphate (cATP) level, UV absorbance at 254 nm (UV254), and total-fluorescence (TF) level may all be used as substitutes to forecast when bacteria will be killed by ozone. The inhibition of four commonly used microbial indicators—total coliforms, Escherichia coli (E. coli), heterotrophic-plate-count (HPC), and faecal coliform is specifically formulated using empirical-linear correlations for log-removal-values (LRV) of TF, UV254, and cATP intensity (R2 = 0.86-0.96) reported by Shi et al. (2022).
Selected Food Application
Food grains
Ozone is a possible fumigant for the management of insect pests in stored products and an active antibacterial ingredient of almost no influence on grain quality (Cullen et al., 2009; Pandiselvam et al., 2017). Grain is treated with ozone typically used in an enclosed storage container with a defined moisture content of grain and fixed thickness of the bed (Ravi et al., 2015). It is important to describe the dynamics of the flow of ozone in gaseous form through different grains to maximize the use of ozone generators in industrial storage containers (Shunmugam et al., 2005; Pandiselvam et al., 2017). Commercially, the innovative Oxygreen is one of the uses of ozone in food grain (Violleau et al., 2012). The Oxygreen technique is a novel method of treating wheat grain with ozone (generated in situ) in an enclosed sequential-batch-process. The Oxygreen technique ensures that the gas and grain come into close, uniform, and regulated contact (DUBOIS et al., 2008). Oxygreen process decreases the amount of mesophilic and coliform bacteria existence on the grains as a result of a factor of 104 cfu/g related to the control process. Compared to the standard milling process, Oxygreen supports in the elimination of smaller-bran particles and middling’s, improving the flavour and potential applications of a number of grain products (DUBOIS et al., 2008). Diffusion of ozone into foodstuffs relies on many intrinsic and external considerations, like as grain temperature, grain moisture content, and bed thickness, design of the processing container, ozone rate of flow, microbial toxicity, ozone intensity and existence of insects (Pandiselvam et al., 2019). Raila et al. (2006) suggested a differential kinetic diffusion equation describe the penetration and mobility of ozone in the grain column.

In this equation Cₒ represent concentration, kg-mol/m3; D represent diffusivity, m2 /s; r represents radius of base of vessel, m; h represents the spices mound height, m; k is factor of ozone absorption; vf is velocity of air seepage in spice layer, m/s; t is the period of interaction to ozone, s.
Pandiselvam et al. (2018) described concentration of ozone in grain bulk by using algebraic equation as given below.

In this equation

and

Where in this equation 2 “c” represent ozone concentration measure in ppm; time express in t in second; De is diffusivity measure in m2s-1, z is ozone velocity in the ‘Z’ direction. Pandiselvam et al. (2015) presented the ozone gas reaction kinetics in organic grains and paddy, correspondingly, by solving equations (3), (4) and (5). They observed that the mechanics of the decomposition of ozone gas followed first order kinetics mode.
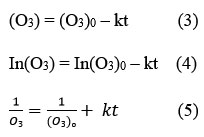
In these equations “( O3)” represents ozone concentration and ( O3)0 is the initial ozone concentration both measure in ppm; time denoted by ‘t’ which is in minute; k is rate of constant (min-1). Ozone has a beneficial result on the baking characteristics of wheat due to increased protein content and, likely, to ozone-promoting premature senescence (Broberg et al., 2015). By using ozone in frame of Oxygreen it is possible to produce better flours to make bread or pastry without the introduction of alpha- amylase, gluten, or ascorbic acid. Treatment of ozone converts 1%-2% of starch to maltose, therefore there is no need to add alpha-amylase. Use of ascorbic acid is also avoided because gluten oxidizes when exposed to ozone. Because as gluten network is wider and broader, wheat of high grade is great abundant and there is usually no need for additional gluten (DUBOIS et al., 2008). The germinating percent test was carried out by equally dispersing twenty-five seeds on every germitest paper, which was utilized in triplicate for every replication of corn. The substrate was moistened/soaked with distilled water in a ratio of 2.5 times the dry paper weight before being placed in a 25 °C germinating chamber reported by Rosa et al. (2022).
![]() |
Figure 1: Schematic depiction of the experimental apparatus used for fumigating |
Fruits and Vegetables
As a daily constitute of diet fruits and vegetables are highly demand in fruit market (Mahajan et al., 2018). Highly perishable nature makes these more vulnerable to mechanical damage which can occur at any time from farm to market. The mechanical damage may lead to further deterioration of fresh produce. Many preservation techniques are utilized to prolong their shelf life and prevent it from contamination. De Oliveira Elias et al. (2018) reported a study to describe the foodborne outbreak linked to fruit and vegetable intake that occurred from 2008 to 2014 in Brazil. Thirty produce associated epidemics caused 2926 infections, 347 hospitalizations, and no loss of life. As etiological agents, only bacterial pathogens were known. Salmonella was the most widespread accounts (30 percent of outbreaks), assisted by Staphylococcus aureus (23.3 percent), Escherichia coli (10 percent), Bacillus cereus (6.6 percent), as well as thermotolerant coliforms (3.3 percent), although other agents of etiology irresolute for 26.6 % of outbreaks. Fruits and vegetables were the most often suspected food vehicles in outbreaks (46.6% of outbreaks). From available preservation technologies, application of ozone is a positive one that is gaining momentum within vegetable and fruit industry. The effectiveness and utility of ozone have been shown over time by its extensive use in water and food treatment (Khadre et al., 2001; Seydim et al., 2004). Vegetables and fruits are a source of microorganisms at harvest time. They will cause severe health problems if not treated before use, and their shelf life is shortened. Ozone is a well-known antimicrobial agent for processing, cleaning, and storing vegetables (Sarron et al., 2021). As an environmentally safe alternate sanitizer, ozone has been used (Alexandre et al., 2012; Karaca, 2011). Tomatoes, apples, and fresh cut lettuce are among the items that have been industrially processed with ozone-containing water. In addition to extending the storage period, ozonation permitted fewer regular adjustments of flume water, resulting in minor maintenance and sewage disposal costs (Strickland et al., 2010; Yaseen et al., 2015; Graham et al., 2011). Ozone in gaseous form is applied to many products includes strawberries, citrus nuts, onions, and potatoes in order to increase their storage life and prevent it from fungal disease spread (Horvitz & Cantalejo, 2014). Providers who used this method were able to improve marketable commodity yields enough to compensate the expense of machinery expenditure throughout the first growing season. Ozone has been used within food industry, either alone or in conjunction with other decontamination methods such as UV light as well as ultrasounds. The use of ozone, UV radiation, and ultrasounds enhances plant hygiene, detoxifies fresh produce prior to, during, and after packing, and thus increases the shelf life of food commodities exiting the plant for dissemination (Steffen et al., 2010).
Ozone and its effect in poultry meat
Innate microbiota was injected into poultry carcasses, that were divided into chest and thigh portions. The injected culture was washed with 3.88 mg/liter ozone at a flowing rate of 2050 mililiters per minute for 20 minutes. The microbial load in the corpse was found to be reduced after the carcass was cleansed with ozone. The life span of chicken meat is extended by lowering the microbial burden about 2.9 log cfu reported by Ayranci et al. (2020). Karamah et al. (2018) demonstrated that ozone levels of 0.21 and 0.38 were capable of disinfecting all aerobic mesophilic microbes with amounts of 0.42 and 0.89 log cfu/g, correspondingly. The water and pH content of the chicken did not change significantly, however the protein amount reduced by 1%.
Ozone’s impact on fresh produce safety
Microorganisms, which include viruses, parasites, fungi/molds and bacteria, are a major source of interest not only because they cause degradation, but also because they have the ability to create mycotoxins. Contamination of fresh produce can be attributed to the inherent microbial-load of resources, poor handling, dissemination, or storage (Karaca & Velioglu, 2007). Unless adequate steps are taken to decontaminate these commodities, their protection can be jeopardized. A measure of effective disinfection must be added before use, in addition to hygienic cultivation techniques, refining, and transportation, as well as proper irrigation with prepared water (Khadre et al., 2001; Glowacz et al., 2015). Fresh produce is generally washed with water or chlorine-solutions that could not be appropriate to eradicate all types of microbes that may be present, specifically in circumstances someplace the microbial load is significant. Sanitizing agents, such as chlorine, hydrogen-peroxide, and ozone, are routinely employed to reduce microbial risk in less treated vegetables. Furthermore, in the existence of organic substances, like as that found in minimally processed vegetables sanitation, hypochlorite interacts with organic-material, generating carcinogenic chemicals which includes trihalomethane and teratogenic-haloacetic-acids, as well as chloroform (de Almeida Lopes et al., 2021). For hygiene purposes, ozonated water has been added to fresh-cut produces, decreasing microbial communities, and extending storage period (Beltrán et al., 2005). Ozone has been routinely utilized in the agricultural industry for washing and storing vegetables and fruits (Joshi et al., 2013). Ozone oxidizes bacteria’s cytoplasmic membranes and cell walls firmly and specifically. Ozonated water has a microbiocidal activity within five seconds of application (Smith & Pillai, 2014). The efficacy of ozone in microbial inactivation or residue removal is highly dependent on factors like as product type, target pathogens, initial biological contents level, bacterial cell physiological condition, ozone physical state. Ozone is an extremely reactive molecule that interacts with virtually any organic and inorganic compound. The expression “residual ozone” refers to the visible ozone level in the treatment media after it has been introduced to the target food substance (Kim et al., 2003). Numerous experiments have shown that ozone is efficient in bacterial inactivation; however, the susceptibility of microorganisms to ozone varies depending on their condition. Application methods that allow for more ozone contact time with product to be handled improve process effectiveness (Aslam, Alam, and Saeed 2020). The microbial-load of trimmed onion-slices was reduced using 3 treatment techniques: splashing, spraying, and dipping reported by (Aslam et al. 2022). Ozone is more effective against bacterial vegetative cells than it is against bacterial spores (Brodowska et al., 2018). It has been proposed that the surface of a cell is the main site of ozonation, with the breakdown of cell envelope unsaturated lipids taking place (Miller et al., 2013). With a substantial portion of the membrane barrier is broken, the cell is disrupted, resulting in cellular content leaking and bacterial cell lysis. When it is insufficient for instantaneous cell death, ozone will enter the bacterium and oxidize some basic constituents such as enzymes, proteins, and nucleic acids. Several experiments have been performed to study the impact of ozone’s effect on food-borne microbes such as Gram positive bacteria (Bacillus cereus, Enterococcus faecalis, Staphylococcus aureus, Listeria monocytogenes) and Gram negative bacteria (Yersinia enterocolitica, Pseudomonas aeruginosa) (Tiwari et al., 2008; Selma et al., 2007; Tzortzakis et al., 2007; Miller et al., 2013). Mycotoxins, secondary metabolites of certain mold species present in both refined and unprocessed foods and feed, harm both human and animal health (Gilbert & Anklam, 2002). Mycotoxin toxicity was the most prevalent form of threat in food products. As a result of aflatoxin exposure, nearly 25% of the world’s crop is destroyed (Karaca & Velioglu, 2007). Fungi like Penicillium and Aspergillus are largely responsible for produce decay. Combined with Fusarium, these fungi are the key sources of mycotoxins, primarily aflatoxins, patulin, and ochratoxin A, which are commonly found fruit juices and dried figs (Freitas-Silva & Venâncio, 2010). Zorlugenç et al. (2008) studied the efficacy of aqueous and gaseous ozone in mold growth inhibition and aflatoxin B1 elimination from dried figs. Ozone is used in industrial applications to eliminate patulin toxin from juices such as apples and pears (Sujayasree et al., 2022). Ozone has been used to inhibit the fungi that causes papaya rot and has also been found to significantly prolong papaya ripening. Residual pesticides which are usually present in fresh produce, since large levels of these compounds are widely used for crop protection. Ecological consequences of pesticide residues existence in the ecosystem are also critical concerns (Wu et al., 2007). Pesticide residues on produce should be eliminated or minimized using successful techniques. Ozone has a long tradition of study in pesticide decay. Many researchers discovered the efficacy of ozone-containing water in the destruction of numerous pesticides in fresh produce (Zhang et al., 2005; Wu et al., 2007). A substantial decline in pesticides has been recorded, which is greatly dependent on temperature and dissolved ozone levels. However, caution must be exercised because ozonation of pesticides can result in byproducts that are potentially more poisonous than the pesticides itself (Ikehata & Gamal El-Din, 2005). Ozone treatment will result in a healthier product with a longer shelf life, the whole consistency of the finished product in terms of physicochemical, nutritional, and sensorial properties must be assured. As a result, process conditions must be considered in such a way that unnecessary losses of quality attributes are avoided as far as possible. Numerous researchers studied the consequence of ozone on the consistency of fresh produce. As with product decontamination, ozone impacts on efficiency are greatly affected by the product. Furthermore, if treatment of ozone is not properly carried out, then it can cause oxidative spoilage of foodstuff.
![]() |
Figure 2: Using gaseous ozone to inactivate foodborne pathogens and their surrogates on fresh produce. |
Utilization of Ozone in Dairy Sector
Good sanitary standards must be implemented in dairy farms in order to produce high quality, microbially safe unpasteurized milk. Considering as ozone would be a potent oxidizing-agent that may kill virus, bacteria, yeast strains, mold, as well as protozoa (Patil et al., 2012). At milk farms, ozone therapy can be used for a number of causes and objectives. After each milking, the tubes that transport milk from separate milking units to a bulk container must be washed. Washing and disinfection methods commonly employ boiled water and chemicals, which uses a lot of energy and chemicals. On dairy farms, the utilization of ozone may significantly reduce chemical expenses and remove the need for boiled water. Kaiser et al. (2013) registered a design for an ozone distribution mechanism, technique, and equipment for cleaning and disinfecting dairy livestock, milking apparatus, and different areas in dairy environments utilizing ozonated water, including ozone at a preferred range of 0.04 to 1.2 parts per million. Ozone has also been utilized to cure cow mastitis, the most expensive and frequent illness within dairy farms. When 6 to 30 milligrams of ozone were injected into each swollen mammary quarter of cattle with severe clinical mastitis, 60 percent of the ill animals cured fully without medication. The efficiency of ozone-treatment was comparable with that of antibiotic-treatment in these animals. The scientists came to the conclusion that ozone treatment is a safe, effective, and low-cost technique to treat mastitis deprived of leaving-antibiotic remains in unprocessed milk (Ogata et al., 2000). Another application of ozone in milk production is the administration of extremely low amounts of ozone into the air within barns to kill airborne germs and remove manure odour. According to chemical oxygen demand (COD) tests, ozonated-water eliminated 84 percent of dairy-residue from dishes, while non-ozonated hot water removed about 51 percent of dairy soil elements, although the both values were not considerably different (P > 0.05). Avila-Sierra et al. (2021) investigated the effectiveness of ozone in removing heat denatured milk protein molecules on stainless steel areas. Whey-protein adsorption was enhanced with both aqueous and gaseous-ozonation. Microorganisms adhering to milk contact surfaces are difficult to eliminate, and it might damage the microbial quality of milk and milk products. Ozonation might be a potential substitute to chlorine-based sanitizers, which are commonly utilized inside the dairy sector. Dosti et al. (2005), during a 10-minute exposure duration, ozonated deionized water that contains 0.5 parts per million ozone reduced the population of major psychrotrophic milk spoilage microorganisms (Alcaligenes faecalis and Pseudomonas fluorescens) on steel plates by almost 4log10 cfu/ml count. The efficiency of ozonated water versus A. faecalis and P. fluorescens biofilms was higher than that of a commercially chlorinated-sanitizer applied at 100 parts per million for 2 minutes. Dosti et al. (2005) found that ozone (0.6 parts per million for 10 minutes) and chlorine (100 parts per million for 2 minutes) significantly decreased the numbers of 3 Pseudomonas species found in biofilms on stainless steel coupon when compared with the control (P <0.05). It’s also noteworthy to mention that combining ozone and powered ultrasound for biofilm eradication is significantly more successful than using either therapy alone (Baumann et al., 2009).
Removing Milk residue and bacteria that produce biofilms on stainless steel surfaces
In addition to eliminate the majority of milk residue from dairy processing apparatus, a rinse with hot water is generally the initial step. Dosti et al. (2005) measured and displayed the performance of ozonated chilled water (10 °C) and hot water (40 °C) as a prerinse for eliminating dairy dirt from steel plates. SEM images revealed that ozonation washed metal parts more effectively than the 40°C hot water-treatment. According the studies of chemical tests, skim milk reduces bacteria population. It was discovered that the therapy reduced the amount of psychrotrophs by greater than 99 percent. Ozonation totally removed Listeria monocytogenes from raw and labelled milk samples, according to Sheelamary & Muthukumar, (2011). In a latest study, the efficacy of ozone treatment for inactivation of microbes in unprocessed milk was examined. Microbial and fungal populations were reported to be reduced by up to 1 log10 cfu when ozone gas was bubbled at 1.5 mg/L for 15 minutes. As a result, ozone action itself was not effective of eliminating a high enough percentage of unprocessed milk bacteria. A Swedish manufacturer has devised a mild procedure that involves pre-ozonation accompanied by a traditional pasteurization stage (Varga et al., 2016). The technique is said to provide commercial liquid milks a longer shelf life while also preventing extensive lipid and protein deterioration in the finished products.
Table 1: Applications of ozone in milk to remove the microorganisms
Application |
treatment |
Targeted substance/microorganism |
Result |
Reference |
Milk residues and biofilm-forming microorganisms are removed from stainless steel surfaces |
For up to 30 minutes, use inside a bath substrate flow apparatus, ozonated water (40 NL/h, 80 g/Nm3) |
Whey-protein concentration (WPC) that has been heat damaged on stainless coupons |
When contrasted to a 0.5 percent NaOH solution, as well as Whey protein concentration removal rates enhanced within 10 minutes |
Jurado-Alameda et al. (2014) |
For 15 minutes, immerse in ozonated cold water (10°C) |
Metal plates with heated dairy soil (20 percent total solids, regenerated non-fat dry milk) |
During subsequent caustic alkali washing, BSA adsorption rates were higher 84 percent of the dairy dirt on plates was eliminated |
Dosti et al. (2005) |
|
Fluid milk |
Gaseous ozone (rate of creation: 0.2 gram per hour) |
Listeria monocytogenes were detected in commercialized raw as well as in branded milk specimens (mean effective counts of 5.5 and 5.7 log10 colony forming units, correspondingly) |
Listeria monocytogenes are completely eliminated after 15 minutes |
Sheelamary and Muthukumar, (2011) |
Ozone gas (1.5 milligrams per liter for 5 to 15 minutes) |
Raw milk microbiota (mean total-plate-count: 4.18 log10 colony-forming units per mL) |
Up to 1 log After 15 minutes, fungal and bacterial counts were reduced by 10 cfu/mL |
Cavalcante et al. (2013a) |
|
Powdered milk products |
ozone gaseous (2 ppb or 32 ppb during production of milk powders) |
Whole Milk Powder and Skim Milk Powder |
Sensitivity decreases as ambient ozone concentrations and fat levels rise |
Kurtz et al. (1969) |
Powdered milk products
Cronobacter spp., commonly classified as Enterobacter sakazakii, have been linked to deadly newborn infections in modern years (Joseph et al., 2011). These bacteria are often identified from powdered milk as well as the surroundings of dried milk processing plants (Torlak et al., 2013). Torlak et al. (2013), demonstrated that ozonation is an efficient approach for killing Cronobacter sakazakii ATCC 51329 cells in milk powders, specifically in dry skim milk. Whole or skimmed powdered milk samples were subjected to gaseous ozone at doses of 2.8 milligrams/L and 5.3 milligrams/L for 0.5 to 2.0 hours. Following 120 minutes of treatment to both ozone concentrations, Cronobacter numbers in skim milk decreased by almost 3log10 cfu/ml. The inclusion of lipid in the dairy product, however, reduced the efficiency of ozonation, as the survivability of C. sakazakii in whole powdered milk was reduced by only 2 log10 cfu/g after 2 hours of gaseous ozonation. Uzun et al. (2012) used gaseous and liquid ozone to cure whey-protein isolate. According to research findings, ozonation significantly improved protein-foaming ability and foam consistency, but also decreased whey-protein dissolvability and emulsion-stability. The dissolvability of protein isolates was reduced more by gaseous ozone applications than by liquid ozonation. Segat et al. (2014) came to the same conclusion, concluding that ozone treatment might be used to generate modified whey-proteins with specified functionality.
Waste water treatment in dairy processing
Water is essential for a variety of processes within dairy sector, including washing, disinfecting, chilling, and heating. As a result, dairy production plants require a lot of freshwater and create a considerable amount of sludge, which has a substantial amount of organic content. (Packyam et al., 2015). Physico-chemical and biological approaches are used to purify dairy waste waters (Beszédes et al., 2009). Moreover, in current era, a number of researchers have investigated the use of ozonation, only or in conjunction with other methods, to recycle, at least to some extent, the wastewater generated by a dairy industry. Beszédes et al. (2015), found ozone processing to be a quite promising strategy for lowering the amounts of organic contaminants in dairy waste-waters in more latest research. Ozone improved the elimination of COD from dairy waste-water samples during following nano-filtration due to its micro-flocculation action. Packyam et al. (2015), utilized a composite phase separation technique for the pre-treatment of a dairy-waste mud with dissolved chemical oxygen demand, suspended particles (SP), and total solids concentration of 400 milligrams/L, 7000 milligrams/L, and 12560 milligrams/L, respectively, in an effort to improve the effectiveness of sludge deterioration. When compared to single pre ozonation, ultrasound mediated o3 pre-treatments usually results in significantly increased chemical oxygen demand (COD) solubilization and suspended solids reduction %, as well as improved the anaerobic decay potential of the milk activated-sludge under optimal working conditions (76.4 kilojoule/TS of particular energy and 0.0011 milligrams O3/milligrams suspended solids). Furthermore, the economic viability of this unique process sono ozone pre-treatment is debatable, as the total cost of (i.e. loss on) a ton of sewage so processed, according the researchers’ estimates, is $4.21. Martins et al. (2010), investigated the use of ozone to oxidize biologically pre-treated cheese-whey waste waters and produce effluents that may be discharged into natural aquatic-systems. The high-organic concentration of cheese-whey was significantly reduced by single ozonation, specifically at an alkaline pH of 10. The addition of 16.5 to 33.0 mM hydrogen per-oxide (H2O2) to the ozone-treatment improved its effectiveness even further. After a sludge bioremediation, it was found that ozonation combination with utilization of H2O2 may be a helpful method for the maximum filtration of cheese-whey industrial effluent, results in final discharges that can be disposed of in water streams.
5 Future Prospective
The efficacy of ozonation is highly dependent on the selection of an appropriate ozone dosage. Because of their high humidity level, phenolic compounds and enzymes, high-moisture foods such as milk, vegetables as well as fruits are particularly impacted by the detrimental properties of ozone (Sandhu et al., 2011). Therefore, for the safe and successful utilization of ozone, the ozone action parameters must be specifically identified for all kinds of food items. In the case of microorganisms, sensitivity to ozone treatment varies depending on the product which was preserved, the initial inoculant level or amount of contaminate, the kind of microorganisms, the physiological condition of the microbial species, and the variety of biological materials. However, if greater ozone levels are necessary for antimicrobial treatments, consideration should be given since it can have a detrimental influence on food quality by decreasing polyphenols, vitamins, and volatile compound levels, as well as loss of hardness and colour changes. Once the safety of a food product has been compromised, the improvement of ozone manufacturing conditions must be evaluated. However, cultivar properties and harvest ripeness are the key elements that define the structure of vegetables and fruits, which in response affect the optimum amount of ozone necessary for treatment. Finally, customer concern about the ozone’s perceived harmful characteristics reduces its acceptability. As a result, extensive information on the usability of ozone-based technologies and their prospective advantages is required to get market approval.
Conclusion
In food industry it is used for the decontamination of product surface and water treatment. It is beneficial for the inactivation of contaminated microorganisms from fruits, vegetables and dry grains. In dairy industry used for the elimination of biofilm producing bacteria and milk residues as well as in cold storage employed to protect the fresh produce from bacteria and mold. This article discusses ozonation as a preservative in dairy, fruits and vegetables, poultry, cereals, and fresh produce in depth, whereas other published articles only discuss one of these groups, making it unique. In the food sector, ozone has shown to be a viable technique, because the usage of ozone does not need extremely high temperatures, it is an energy-saving technique. Furthermore, because ozone is created insitu, the storing expenses of decontaminators are reduced when using this technology. The ideal ozone concentration, contact duration, and other treatment parameters should be established for all goods for efficient and secure usage in food processing. Because every ozone application is different, pilot tests must be completed before beginning commercial application.
Acknowledgements
Authors are thankful to Government College University for providing literature collection facilities
Conflict of Interest
Authors declare that they have no conflict of interest.
Funding Sources
The authors declare that no funds, grants, or other support were received during the preparation of this manuscript.
References
- Artes F., Allende A. Minimal fresh processing of vegetables, fruits and juices. In Emerging technologies for food processing . 2005;677-716. Academic Press. https://doi.org/10.1016/B978-012676757-5/50028-1
- Aslam R., Alam M S., Saeed. P. A. Sanitization Potential of Ozone and Its Role in Postharvest Quality Management of Fruits and Vegetables. Food Engineering Reviews. 2020;12 (1): 48–67. doi:https://doi.org/10.1007/s12393-019-09204-0.
- Aslam R., Alam M. S., Pandiselvam R. Aqueous ozone sanitization system for fresh produce: Design, development, and optimization of process parameters for minimally processed onion. Ozone: Science & Engineering. 2022; 44(1):3-16.
- Ayranci U. G., Ozunlu O., Ergezer H., Karaca H. Effects of ozone treatment on microbiological quality and physicochemical properties of turkey breast meat. Ozone: Science & Engineering. 2020: 42(1); 95–103. https://doi.org/10.1080/01919512.2019.1653168
- Avila-Sierra A., Vicaria J. M., Lechuga M., Martínez-Gallegos J. F., Olivares-Arias V., Medina-Rodríguez, A. C., … Jurado-Alameda, E. Insights into the optimization of the Clean-In-Place technique: Cleaning, disinfection, and reduced environmental impact using ozone-based formulations. Food and Bioproducts Processing. 2021; 129:124-133. https://doi.org/10.1016/j.fbp.2021.08.003
- Beltrán D., Selma M. V., Marín A., Gil, M. I. Ozonated water extends the shelf life of fresh-cut lettuce. Journal of agricultural and food chemistry. 2005; 53(14):5654-5663.https://doi.org/ 10.1021/acs.jafc. 7b01667
- Beszédes S., Kertész S., László Z., Szabo G., Hodur, C. Biogas production of ozone and/or microwave-pretreated canned maize production sludge. Ozone: science & engineering. 2009;31(3):257-261. https://doi.org/10.1080/01919510902841218
- Broberg M. C., Feng Z., Xin Y., Pleijel H. Ozone effects on wheat grain quality–A summary. Environmental Pollution. 2015;197:203-213. https://doi.org/10.1016/j.envpol.2014.12.009
- Brodowska A. J., Nowak, A., Śmigielski, K. Ozone in the food industry: Principles of ozone treatment, mechanisms of action, and applications: An overview. Critical reviews in food science and nutrition. 2018;58(13):2176-2201. https://doi.org/10.1080/10408398. 2017.1308313
- Cavalcante M. A., Leite Júnior B. D. C., Tribst A. A. L., Cristianini, M. Improvement of the raw milk microbiological quality by ozone treatment. International Food Research Journal, 2013; 20(4).
- Choi M. R., Liu Q., Lee S. Y., Jin J. H., Ryu S., Kang, D. H. Inactivation of Escherichia coli O157: H7, Salmonella typhimurium and Listeria monocytogenes in apple juice with gaseous ozone. Food Microbiology. 2012;32(1):191–195.
- Cullen P. J., Norton T. Ozone sanitisation in the food industry. In Ozone in Food Processing. 2012; 63– 176. C O’Donnell, B K Tiwari, P J Cullen and R G Rice, eds. Oxford: Wiley-Blackwell.
- de Almeida Lopes M. M., de Lucena H. H., da Silveira M. R. S., dos Santos Garruti D., Machado T. F., de Aragão F. A. S., de Oliveira Silva, E. The use of electrolyzed water as a disinfectant for fresh cut mango. Scientia Horticulturae, 2021;287:110227.
- de Oliveira Elias S., Tombini Decol L., Tondo, E. C. Foodborne outbreaks in Brazil associated with fruits and vegetables: 2008 through 2014. Food Quality and Safety. 2018; 2(4): 173-181. https://doi.org/10.1093/fqsafe/fyy022
- de Souza L. P., Faroni L. R. D. A., Heleno F. F., Cecon P. R., Gonçalves T. D. C., da Silva G. J., Prates L. H. F. Effects of ozone treatment on postharvest carrot quality. Lwt. 2018; 90:53-60.
- Dosti B., Guzel-seydim, Z. E. Y. N. E. P., Greene, A. K. Effectiveness of ozone, heat and chlorine for destroying common food spoilage bacteria in synthetic media and biofilms. International journal of dairy technology. 2005;58(1):19-24. https://doi.org/10.1111/j.1471-0307.2005.00176.x
- Dubois M., Pernot A. G., Coste, C. The Oxygreen® Process, a New Tool for Global Improvement of Whole Wheat Grain and of All Products from Wheat Grain Milling. In Consumer Driven Cereal Innovation .2008:121-124. https://doi.org/10.1016/B978-1-891127-61-8.50030-3
- Freitas-Silva O., Venâncio, A. Ozone applications to prevent and degrade mycotoxins: a review. Drug metabolism reviews. 2010;42(4): 612-620. https://doi.org/10.3109/03602532.2010.484461
- Gilbert J., Anklam, E. Validation of analytical methods for determining mycotoxins in foodstuffs. TrAC Trends in Analytical Chemistry. 2002;21(6-7):468-486. https://doi.org/10.1016/S0165-9936(02)00604-0
- Glocker E. O., Kotlarz D., Boztug K., Gertz E. M., Schäffer A. A., Noyan F., … & Klein C. Inflammatory bowel disease and mutations affecting the interleukin-10 receptor. New England Journal of Medicine, 2009;361(21):2033-2045. DOI: 10.1056/NEJMoa0907206
- Glowacz M., Colgan R., Rees, D. The use of ozone to extend the shelf‐life and maintain quality of fresh produce. Journal of the Science of Food and Agriculture. 2015; 95(4):662-671. https://doi.org/10.1002/ jsfa.6776
- Gottschalk C., Libra J. A., Saupe, A. Ozonation of water and waste water: A practical guide to understanding ozone and its applications. 2009. John Wiley & Sons.
- Graham T., Zhang P., Woyzbun E., Dixon, M. Response of hydroponic tomato to daily applications of aqueous ozone via drip irrigation. Scientia Horticulturae. 2011;129(3):464-471. https://doi.org/10.1016/ j.scienta.2011.04.019
- Guzel-Seydim Z. B., Greene A. K., Seydim, A. C. Use of ozone in the food industry. LWT-Food Science and Technology. 2004;37(4): 453-460. https://doi.org/10.1016/j.lwt.2003.10.014
- Horvitz S., Cantalejo, M. J. Application of ozone for the postharvest treatment of fruits and vegetables. Critical reviews in food science and nutrition. 2014;54(3):312-339. https://doi.org/10.1080/10408398.2011.584353
- Ikehata K., Gamal El-Din M. Aqueous pesticide degradation by ozonation and ozone-based advanced oxidation processes: a review (Part I). Ozone: science and engineering. (2005). 27(2), 83-114. https://doi.org/10.1080/01919510590925220
- Islam F., Saeed F., Afzaal M., Ahmad A., Hussain M., Khalid M. A., Saewan S. A., Khashroum A. O. Applications of green technologies based approaches for food safety enhancement: A comprehensive review. Food Science & Nutrition. 2022;00:1–13.
- Joseph S., Forsythe, S. J. Predominance of Cronobacter sakazakii sequence type 4 in neonatal infections. Emerging infectious diseases. 2011; 17(9):1713. doi: 10.3201/eid1709.110260
- Joshi K., Mahendra R., Alagusundaram K., Norton T., Tiwari B. K. Novel disinfectants for fresh produce. Trends in Food Science & Technology. 2013;34(1):54-61.
- Jurado-Alameda E., Altmajer-Vaz D., García-Román M., Jiménez-Pérez J. L. Study of heat-denatured whey protein removal from stainless steel surfaces in clean-in-place systems. International Dairy Journal. 2014;38(2):195-198. https://doi.org/10.1016/j.idairyj.2014.01.006
- Jaramillo-Sánchez G., Contigiani E. V., Castro M. A., Hodara K., Alzamora S. M., Loredo A. G., Nieto, A. B. Freshness maintenance of blueberries (Vaccinium corymbosum L.) during postharvest using ozone in aqueous phase: Microbiological, structure, and mechanical issues. Food and Bioprocess Technology. 2019;12(12):2136–2147.
- Kaiser H., Sanchez C., Heacox S., Kathriner A., Ribeiro A. V., Soares Z. A., … & O’Shea M. First report on the herpetofauna of Ataúro Island, Timor Leste. Check List. 2013; 9(4):752-762. https://doi.org/ 10.15560/9.4.752
- Karaca H. Use of ozone in the citrus industry. Ozone: Science & Engineering. 2010; 32(2), 122-129. https://doi.org/10.1080/ 01919510903520605
- Karaca H., Velioglu Y. S. Ozone applications in fruit and vegetable processing. Food Reviews International. 2007;23(1):91-106. https://doi.org/10.1080/87559120600998221
- Khadre M. A., Yousef A. E., Kim J. G. Microbiological aspects of ozone applications in food: a review. Journal of food science. 2001;66(9):1242-1252. https://doi.org/10.1111/j.1365-2621.2001.tb15196.x
- Khadre M. A., Yousef A. E., Kim J. G. Microbiological aspects of ozone applications in food: a review. Journal of food science. 2001;66(9):1242-1252. https://doi.org/10.1016/S1043-4526(03)45005-5
- Kurtz F. E., Tamsma A., Selman R. L., Pallansch, M. J. Effect of pollution of air with ozone on flavor of spray-dried milks. Journal of Dairy Science. 1969;52(2):158-161.
- Li M. M., Guan E. Q., Bian K. Effect of ozone treatment on deoxynivalenol and quality evaluation of ozonized wheat. Food Additives & Contaminants: Part A. 2015;32(4):544-553. https://doi.org/10.1080/19440049.2014.976596
- Kurtz F. E., Tamsma A., Selman R. L., Pallansch, M. J. Effect of pollution of air with ozone on flavor of spray-dried milks. Journal of Dairy Science. 1969;52(2):158-161.
- Li M. M., Guan E. Q., Bian K. Effect of ozone treatment on deoxynivalenol and quality evaluation of ozonized wheat. Food Additives & Contaminants: Part A. 2015;32(4):544-553.
- Mahajan B. C., Tandon R., Kapoor S., Sidhu M. K. Natural coatings for shelf-life enhancement and quality maintenance of fresh fruits and vegetables—A review. J. Postharvest Technol. 2018:6(1);12-26. https://doi.org/10.22271/tpi.2021.v10.i4q.6176
- Manousaridis G., Nerantzaki A., Paleologos E. K., Tsiotsias A., Savvaidis I. N., Kontominas M. G. Effect of ozone on microbial, chemical and sensory attributes of shucked mussels. Food microbiology. 2005;22(1):1-9. doi:10.1016/j.fm.2004.06.003
- Martins R. C., Quinta-Ferreira R. M. Final remediation of post-biological treated milk whey wastewater by ozone. International Journal of Chemical Reactor Engineering. 2010;8(1).
- Miller F. A., Silva C. L., Brandão T. R. A review on ozone-based treatments for fruit and vegetables preservation. Food Engineering Reviews. 2013;5(2):77-106. doi; 10.1007/s12393-013-9064-5
- Mishra G., et al. Disinfestation of stored wheat grain infested with Rhyzopertha dominica by ozone treatment: process optimization and impact on grain properties.Journal of the Science of Food and Agriculture .2019;99.11: 5008-5018.
- Mohamed M H., Ammar M A M., Zaki Z M., Youssef A E K. Ozone as a Solution for Eliminating the Risk of Aflatoxins Detected in Some Meat Products. Curr Res Nutr Food Sci .2021; 10(1). doi : http://dx.doi.org/ 10.12944/CRNFSJ.10.1.28
- O’Donnell C., Tiwari B. K., Cullen P. J., Rice R. G. (Eds.). Ozone in food processing. 2012. John Wiley & Sons.
- Ogata A., Nagahata H. Intramammary application of ozone therapy to acute clinical mastitis in dairy cows. Journal of Veterinary Medical Science. 2000;62(7):681-686.
- Ogunsona E. O., Muthura R., Ojogbo E., Valerio O., Mekonnen, T. H. Engineered nanomaterials for antimicrobial applications: A review. Applied Materials Today. 2020;18:100473. 10.1016/j.apmt.2019.100473
- Packyam G. S., Kavitha S., Kumar S. A., Kaliappan S., Yeom I. T., Banu J. R. Effect of sonically induced deflocculation on the efficiency of ozone mediated partial sludge disintegration for improved production of biogas. Ultrasonics sonochemistry. 2015;26:241-248. https://doi.org/10.1016/j.ultsonch.2015.01.015
- Paes J. L., Faroni L. R., Martins M. A., Cecon P. R., Heleno F. F. Reaction kinetics of ozone gas in wheat flour. Engenharia Agrícola. 2017;37:520-528. https://doi.org/10.1590/1981-6723.02217
- Pandiselvam R., Chandrasekar V., Thirupathi, V. Numerical simulation of ozone concentration profile and flow characteristics in paddy bulks. Pest management science.2017:73(8);1698-1702. DOI 10.1002/ps.4516
- Pandiselvam R., Subhashini S., Banuu Priya E. P., Kothakota A., Ramesh S. V., Shahir, S. Ozone based food preservation: a promising green technology for enhanced food safety. Ozone: Science & Engineering. 2019;41(1):7-34. https://doi.org/10.1080/01919512. 2018.1490636
- Pandiselvam R., Thirupathi V., Anandakumar S. Reaction kinetics of ozone gas in paddy grains. Journal of Food Process Engineering. 2015;38(6):594-600. doi:10.1111/jfpe.12189
- Pandiselvam R., Thirupathi V., Chandrasekar V., Kothakota A., Anandakumar S. Numerical simulation and validation of mass transfer process of ozone gas in rice grain bulks. Ozone: Science & Engineering. 2018;40(3):191-197. https://doi.org/10.1080/01919512.2017.1404902
- Pandiselvam R., Prithviraj V., Kothakota A., Prabha K. Ozone Processing of Foods: Methods and Procedures Related to Process Parameters. In Emerging Food Processing Technologies. Humana, New York. 2022:59-75.
- Patil S., Bourke P. Ozone processing of fluid foods. In Novel thermal and non-thermal technologies for fluid foods. Academic Press. 2012: 225-261. https://doi.org/10.1016/B978-0-12-381470-8.00009-8
- Panebianco F., Rubiola S., Chiesa F., Civera T., Di Ciccio P. A. Effect of gaseous ozone treatment on biofilm of dairy-isolated Pseudomonas spp. strains. Italian Journal of Food Safety. 2022:11(2).
- Perry J. J., Peña-Melendez M., Yousef A. E. Ozone-based treatments for inactivation of Salmonella enterica in tree nuts: Inoculation protocol and surrogate suitability considerations. International journal of food microbiology. 2019;297:21-26.
- Prabha V.I.T.H.U., Barma R.D., Singh R., Madan, A. Ozone technology in food processing: A review. 2015. https://doi.org/10.1590/1981-6723.02217
- Raila A., Lugauskas A., Steponavicius D., Railiene M., Steponaviciene A., Zvicevicius E. Application of ozone for reduction of mycological infection in wheat grain. Annals of Agricultural and Environmental Medicine. 2006 :13(2), 287-294.
- Ramos B., Miller F. A., Brandão T.R.S., Teixeira P., Silva C.L.M. Fresh fruits and vegetables—an overview on applied methodologies to improve its quality and safety. Innovative Food Science & Emerging Technologies. 2013;20:1-15. https://doi.org/10.1016/j.ifset.2013.07.002
- Ravi P., Venkatachalam T., Rajamani M. Decay rate kinetics of ozone gas in rice grains. Ozone: Science & Engineering. 2015;37(5):450-455. https://doi.org/10.1080/01919512. 2015.1040912
- Rosa C. C., De Alencar E. R., Souza N. O. S., Bastos C. S., Suinaga F. A., Ferreira W. F. D. S. Physiological Quality of Corn Seeds Treated with Gaseous Ozone. Ozone: Science & Engineering. 2022;44(1):117-126.
- Rice R. G., Graham D. M., Lowe M. T. Recent ozone applications in food processing and sanitation. Food Safety Magazine. 2002;8(5):10-17.
- Rekhate C. V., Srivastava J. K. Recent advances in ozone-based advanced oxidation processes for treatment of wastewater-A review. Chemical Engineering Journal Advances. 2020;3:100031.
- Sandhu H. P., Manthey F. A., Simsek S. Quality of bread made from ozonated wheat (Triticum aestivum L.) flour. Journal of the Science of Food and Agriculture. 2011;91(9):1576-1584. https://doi.org/10.1080/ 19476337.2015.1133714
- Sarron E., Gadonna-Widehem P., Aussenac T. Ozone Treatments for Preserving Fresh Vegetables Quality: A Critical Review. Foods. 2021;10(3):605.
- Smith J. S., Pillai S. Irradiation and Food Safety. Food Technology (Chicago). 2004;58(11):48–55.
- Segat A., Misra N. N., Fabbro A., Buchini F., Lippe G., Cullen P. J., Innocente N. Effects of ozone processing on chemical, structural and functional properties of whey protein isolate. Food Research International. 2014;66:365-372. https://doi.org/10.1016/ j.foodres.2014.10.002
- Selma M. V., Beltrán D., Allende A., Chacón-Vera E., Gil M. I. Elimination by ozone of Shigella sonnei in shredded lettuce and water. Food microbiology. 2007;24(5):492-499. https://doi.org/10.1016/ j.fm.2006.09.005
- Shi Q., Chen Z., Wei F., Mao Y., Xu Q., Li,K., … & Hu H. Y. Identification of surrogates for rapid monitoring of microbial inactivation by ozone for water reuse: A pilot-scale study. Journal of Hazardous Materials. 2022;424:127567.
- Sheelamary M., Muthukumar M. Effectiveness of ozone in inactivating Listeria monocytogenes from milk samples. World Journal of Young Researchers. 2011;1(3):40-44.
- Sheelamary M., Muthukumar M. Effectiveness of ozone in inactivating Listeria monocytogenes from milk samples. World Journal of Young Researchers. 2011;1(3):40-44. http://www.rrpjournals.com /uniqueness. html
- Shunmugam G., Jayas D. S., White N. D. G., Muir W. E. Diffusion of carbon dioxide through grain bulks. Journal of Stored Products Research. 2005; 41(2) :131-144. https://doi.org/10.1016/j.jspr.2003.09.005
- Smith J. S., Pillai S. Irradiation and food safety. Food technology (Chicago). 2004;58(11):48-55. https://doi.org/10.1590/1981-6723.02217
- Steffen H., Rice R. G. New restaurant concept relies on ozone, UV radiation, ultrasound and modified air packaging. Ozone: Science & Engineering. 2010;32(2):137-143. https://doi.org/10.1080/ 01919510903489561
- Sujayasree O. J., Chaitanya A. K., Bhoite R., Pandiselvam R., Kothakota A., Gavahian M., Mousavi Khaneghah, A. Ozone: An advanced oxidation technology to enhance sustainable food consumption through mycotoxin degradation. Ozone: Science & Engineering. 2022;44(1):17-37.
- Strickland W., Sopher C. D., Rice R. G., Battles G. T. Six years of ozone processing of fresh cut salad mixes. Ozone: science & engineering. 2010;32(1):66-70. https://doi.org/10.1080/01919510903489355
- Tiwari B. K., Brennan C. S., Curran T., Gallagher E., Cullen P. J., O’Donnell C. P. Application of ozone in grain processing. Journal of cereal science. 2010;51(3):248-255. https://doi.org/10.1016/j.jcs.2010.01. 007
- Tiwari B. K., Muthukumarappan K., O’Donnell C. P., Cullen P. J. Kinetics of freshly squeezed orange juice quality changes during ozone processing. Journal of agricultural and food chemistry. 2008;56(15):6416-6422. https://doi.org/10.1021/jf800515e
- Torlak E., Sert D. Inactivation of Cronobacter by gaseous ozone in milk powders with different fat contents. International Dairy Journal. 2013; 32(2):121-125. https://doi.org/10.1016/j.idairyj.2013.05.013
- Tzortzakis N., Borland A., Singleton I., Barnes J. Impact of atmospheric ozone-enrichment on quality-related attributes of tomato fruit. Postharvest Biology and Technology. 2007;45(3):317-325. https://doi.org/10.1016/j.postharvbio.2007.03.004
- Uzun H., Ibanoglu E., Catal H., Ibanoglu S. Effects of ozone on functional properties of proteins. Food Chemistry. 2012;134(2):647-654. https://doi.org/10.1016/j.foodchem.2012.02.146
- USFDA. United States Food and Drug Administration. Full Text of the Food Safety Modernization Act (FSMA). 2011. 111th Congress Public Law 353. https://www.fda.gov/food/food-safety-modernization-act-fsma/full-text-food-safety-modernization-act-fsma
- van Leeuwen J., Sridhar A., Harrata A.K., Esplugas M., Onuki S., Cai L., Koziel J.A. Improving the Biodegradation of Organic Pollutants with Ozonation during Biological Wastewater Treatment. Ozone Sci. Eng. 2009;31: 63–70.
- Varga L., Szigeti J. Use of ozone in the dairy industry: A review. International Journal of Dairy Technology. 2016;69(2):157-168. https://doi.org/10.1111/1471-0307.12302
- Violleau F., Pernot A. G., Surel O. Effect of Oxygreen® wheat ozonation process on bread dough quality and protein solubility. Journal of Cereal Science. 2012;55(3):392-396. https://doi.org/10.1016/j.jcs.2012. 01.014
- Wu J. G., Luan T. G., Lan C. Y., Lo W. H., Chan G. Y. S. Efficacy evaluation of low-concentration of ozonated water in removal of residual diazinon, parathion, methyl-parathion and cypermethrin on vegetable. Journal of Food Engineering. 2007;79(3):803-809. https://doi.org/10.1016/j.jfoodeng.2006.02.044
- Yaseen T., Ricelli A., Turan B., Albanese P., D’onghia A. M. Ozone for post-harvest treatment of apple fruits. Phytopathologia Mediterranea. 2015;94-103.
- Zhang L., Lu, Z., Yu, Z., Gao, X. Preservation of fresh-cut celery by treatment of ozonated water. Food control. 2005;16(3):279-283. https://doi.org/10.1016/j.foodcont.2004.03.007
- Zhou Z., Zuber S., Cantergiani F., Sampers I., Devlieghere F., Uyttendaele M. Inactivation of foodborne pathogens and their surrogates on fresh and frozen strawberries using gaseous ozone. Frontiers in Sustainable Food Systems. 2018;2:51.
- Zorlugenç B., Zorlugenç F. K., Öztekin S., Evliya I. B. The influence of gaseous ozone and ozonated water on microbial flora and degradation of aflatoxin B1 in dried figs. Food and chemical toxicology. 2008;46(12):3593-3597. https://doi.org/10.1016/j.fct.2008.09.003

This work is licensed under a Creative Commons Attribution 4.0 International License.