Introduction
Extending the shelf life of foods such as fruits and vegetables is one of the main objectives of the food industry and dehydration is one of the most effective methods, since it allows slowing the growth of microorganisms and their deterioration by eliminating free water from the food. Drying is the most widely used dehydration method; however, the use of high temperatures tends to decrease the quality of food by generating browning in the tissue and reducing its nutritional value due to the use of high temperatures for long periods of time. 1–3
Faced with this drying problem, osmotic dehydration is a great alternative, as its use allows dehydrating foods without incurring high energy costs, significant loss of nutrients, or deterioration of sensory characteristics. 4 The method consists of partial dehydration by immersing pieces of food in highly concentrated or hypertonic solutions.5 So the loss of water is the product of the formation of a pressure differential between the osmotic solution and the membranes that are part of the parenchymal tissue of fruits.6 It has been demonstrated that osmotic dehydration can reduce the moisture content of fruits and vegetables by up to 50-60%,7 hence this method is used as a pretreatment for drying, since a considerable percentage of water in the food has been reduced, the drying time and exposure to heat are significantly reduced, thus reducing the damage caused by high temperatures, obtaining highly stable products over time and with lower nutritional and sensory losses.
On the other hand, when evaluating preservation processes such as dehydration, it is done by measuring and controlling the variables to be analysed, using laboratory equipment such as thermometers, refractometers, scales, etc., which require the constant presence of an evaluator.8 Although process automation is already a reality, nowadays the digitization of the food industry or also known as “Food Industry 4.0” is being sought, which includes the use of IoT (Internet of Things) as a main component for its development,9,10 which would allow remote monitoring and control of processes without the need for intervention in them. According to Dadhaneeya et al. 11 in the last ten years the use and development of IoT in the food industry has grown rapidly and extensively. For example, this technology has been used in processes such as solar dehydration to monitor and control process parameters;12,13 on the other hand, in hot air-drying processes, IoT has been used to control, alert and monitor process parameters remotely.14,15 Another process implemented with IoT is the wine industry where it is used to monitor and control fermentation16,17 and has even been used throughout the production chain, from monitoring the state of the fields, production control to storage.18 And so it is possible to name several processes where IoT has been used: meat industry,19,20 canning,21 brewing,22,23 among others11,24. Taking into account the above, it can be seen the high interest of the food industry globally in implementing this technology, so it is now necessary to follow the tendency regarding the use of IoT in the design of equipment for the food industry, not only as an industrial trend but also as a method of improving process control.
However, even though the osmotic dehydration process has been studied by many authors and there are works in which part of the process has been designed and automated,25–28 there are still no data on the use of the Internet of Things (IoT) for remote monitoring of process variables, let alone any system that allows knowing in real time the change in the concentration of medium osmotic solution, since the dilution is a factor that can negatively affect osmodehydration.
Materials and Methods
The first step in designing the prototype was to determine the measurable variables involved in the process, such as the concentration, temperature, and agitation of the osmotic solution. Several authors cited in Ahmed et al.5 point out that high sucrose concentrations generate a higher osmotic pressure, which leads to higher rates of water loss and solids gain during treatment. research carried out in this process evaluates concentrations ranging from 30 to 60 °Brix, 29–32 since higher concentrations would lead to a very high solids gain, which is not exactly desirable during the process. For temperature, a similar behaviour occurs with concentration, as increasing this factor reduces the viscosity of the syrup and improves mass transfer.33 However, it is advisable to work with temperatures below 50°C, as higher temperatures favour the volatilisation of some nutrients and damage to the feed tissue.34 On the other hand, the agitation is important to avoid the formation of concentration and temperature gradients in a liquid medium.35
Taking into account the above-mentioned in the previous paragraph, the ranges in which the factors will oscillate were determined; concentration of the osmotic solution: 45 – 60 °Brix, temperature: 45 – 50 °C and agitation. After that, the existence of sensors or actuators to measure and control these factors was identified; this phase was important to determine the suitability of these components. Furthermore, the design was carried out using the AutoCAD software.
Prototype design
The prototype consists of a stainless-steel tank (see Figure 1), with a capacity of 18 liters. Sstainless-steel is a material widely used in the construction of equipment for the food industry, due to its durability and resistance to corrosion.36 The capacity of the tank was calculated taking into consideration the amount of fruit to be processed in the equipment, and the proportion of fruit:osmotic solution to be considered in the process.
![]() |
Figure 1: Osmodehydrator Tank. |
On the other hand, the fruit: solution ratio plays an important role during the OD process, since high concentrations need to be maintained for the process to be effective, so a higher fruit: syrup ratio is desirable. Ratios from 1:2 to 1:30 have been studied, however it is recommended to use ratios of 1:4 to 1:6 due to process economy37. So, the ratio chosen for the prototype was 1:4, being the volume of osmotic solution to be used 10 litres for every 2.5 Kg of processed fruit
The volume occupied by the fruit was calculated from the density and mass of the fruit (Equation 1). For this purpose, Sweat38,39, who studied the densities of some of the most demanded fruits, identified that their densities ranged from 0.840 kg/m3 to 1.422 kg/m3. These data allowed the design of a prototype that could be used to process different varieties of fruit. In view of this, the lowest density found in this study will be taken into account for the calculation of the volume occupied by the fruit, in order to determine the maximum volume that a fruit can occupy.
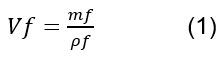
From Equation 1, where Vf is the volume of the fruit, mf is the mass and ρ is the density; the following volume occupied by the fruit was obtained:

So, the volume of the syrup plus the fruit will be:

However, according to Deza et al.27 a safety factor equivalent to 25% should be taken into account, due to the volume variations that can arise with agitation. Although the equipment does not use agitation, it was considered to build a basket that allows for the buoyancy of the tilt sensor (see Figure 2). This will prevent the fruit from accumulating around the sensor, thus ensuring that it floats freely and does not give erroneous values. Taking this into account it was determined that the safety volume (vs) to be increased will be 3.6 L (Equation 4).

![]() |
Figure 2: Basket designed for float sensor. |
Thus, the total volume was obtained by adding the volume occupied by the fruit:osmotic solution system plus the safety volume.

The design also includes a mobile metal structure (See figure 3). All components of the equipment will be assembled on this structure. It also has wheels to generate easy mobility to the prototype.
![]() |
Figure 3: Metal support structure. |
Temperature Control
To measure the temperature of the osmotic solution during the process, a bulb terminal temperature sensor was used, since this will be used to build a thermostat to control the temperature. A heating resistor was used, to generate the heating of the osmotic solution depending on the set point established in the thermostat.
Concentration monitoring
The Tilt Float was used (See figure 4), which is a wireless hydrometer that allows specific gravity and temperature readings in real time using devices such as Android phones, iPhone/iPad compatible with the Tilt application, also the data collected by this sensor can be saved in the cloud, using a free Google Spreadsheet template or third-party clouds.40
The tilt-float allows for specific gravity readings in the range of 1,190 to 1,310, which is equivalent to 42.2 and 63.7 ° Brix. This device works with Bluetooth and its system is based on a floating cylinder in which the only external reference that affects it is gravity. The angle of flotation will change with floatability, which is influenced by the density of the liquid and, as a consequence, the sugar content.41
![]() |
Figure 4: Tilt Hydrometer. (a) Tilt picture. |
Agitation Control
Agitation will be carried out using a recirculation system (see Figure 5) to prevent the use of agitator blades from damaging the fruit and hindering the use of the basket.
![]() |
Figure 5: Diagram of the agitation system by recirculation. The system will operate with two water pumps and control valves. |
Materials and methods to implement IoT
To implement the Internet of Things (IoT), a three-tier or layered architecture was used. This architecture is one of the simplest and comprises a sensing layer, network layer and application layer. Figure 6 shows the schematic of the architecture used to implement the IoT in our prototype.
![]() |
Figure 6: Three-layer architecture for IoT |
The reception layer or also called sensor layer is responsible for detecting and collecting the information obtained from the sensors, converting them into digital signals which will be transmitted to the network layer.42 To carry out this layer, the Tilt Hydrometer temperature and density sensor was used. This sensor facilitates access to the second network layer, as it employs Bluethooth as a means of data transmission and processing. In the IoT domain, Bluethooth is one of the four most popular IoT protocols, along with WiFi, LoRa, and Zigbee.43 To implement the third layer of the IoT application, a compatible application called Tilt2 was used, which allows obtaining temperature and specific gravity data in real time, as well as generate graphs and tables for a better analysis of the process.
It is worth mentioning that the implementation of IoT in this equipment provides great benefits during the process, since it is possible to obtain data on its status in real time and remotely, without the need to be close to the equipment or use various measuring equipment such as refractometers and thermometers. This permanent monitoring of the process also allows a preventive analysis of the data obtained, so that the operator can make decisions regarding the process. On the other hand, currently implementing IoT is not a difficult task since in recent years smart sensors have been created that facilitate access to the sensed data using the internet. However, the implementation process can be a bit costly, since many of the smart sensors tend to have high prices, due to the wide possibilities of connection they have and the high precision they possess.44 But this is where the long-term benefit of implementation comes in, since the process is reduced to the use of a single sensor, leaving aside the acquisition of measuring equipment that requires the presence of an operator for its use, in addition it reduces the time for measurement and decision making during the process, reduces costs by being able to detect failures in time and act before they imply a double or triple expense.
Evaluation of equipment performance
The operation of the prototype was tested on two fruits: apple and melon. The methodology for the osmotic dehydration process is shown in Figure 7.
![]() |
Figure 7: Flow chart of the Osmotic Dehydration Process |
Estimation of weight loss, water loss and solids gain
With the data of initial weight of the fruit ( M0), weight at the end of the process ( Mf), degrees °Brix before treatment (S0 ) and after the process ( Sf), the calculations of initial moisture of the fruit (H0 ) and after the process (Hf); we proceeded to calculate the weight reduction (WR), water loss (WL) and solids gain (SG) according to the following equations:
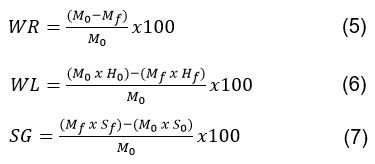
Statistical analysis
A completely randomised design (CRD) with a 2Ax2B factorial arrangement was used. The factors studied were concentration and temperature, where the levels of the sucrose syrup concentration factor were 45°Brix and 60°Brix; while for temperature they were 35°C and 45°C. Four treatment combinations were obtained as shown in table 5. The response variables were weight loss (WR), water loss (WL) and solids gain (SG).
For each treatment, two replicates were run with a duration of 3 hours for each test. The data obtained were evaluated with the Shapiro-wilk normality test where a p> 0.05 was obtained, indicating compliance with the assumptions of normality, which allowed an analysis of variance (ANOVA) to be carried out. The statistically significant effect of the parameters was determined with Tukey’s test.
Results and discussion
The built equipment has a cylindrical tank design with a valve-driven recirculation system, temperature control, and syrup concentration monitoring. As well as a control box that will allow to monitor the status of the variables evaluated during the process. Regarding the IoT, the equipment is equipped with a Tablet, which allows monitoring the temperature and concentration data in real time, through the use of the wireless sensor Tilt Hydrometer. The design incorporates ergonomic features for the displacement of the equipment through the use of a bearing system. The equipment has overall height dimensions of 1.65 m and a width of 48.5 cm.
![]() |
Figure 8: Osmotic dehydration equipment with IoT. (a)Osmodehydrator tank. (b)Recirculation hose. (c)Water pumps. (d)Stopcocks for pump 1. |
Tests on fruits
Weight reduction (WR) and water loss (WL)
After analysing the results obtained from the osmodehydration treatment at the end of the three hours of processing, the existence of significance between the factors and their interaction was demonstrated with a p value <0.05. The greatest weight loss for both fruits was observed with treatments carried out at concentrations of 60°Brix, reaching values of 66.028% and 75.259% for melon and 37.448% and 44.007% for apple, the only difference between them being the use of temperatures of 35 ° C for the lowest losses and 45 ° C for the highest losses (See Table 1).
Table 1: Percentage weight loss during osmotic dehydration of melon and apple.
Factor A: Concentration |
Factor B: Temperature |
||
35 °C |
45 °C |
||
Melon |
45 °Brix |
58.066 ± 0.138b |
55.298 ± 0.278a |
60 °Brix |
66.028 ± 0.430c |
75.259 ± 0.351d |
|
Apple |
45 °Brix |
30.965 ± 0.151a |
32.281 ± 0.143b |
60 °Brix |
37.448 ± 0.173c |
44.007 ± 0.131d |
Figure 9 shows the interaction graphs of the factors with respect to weight loss in melon and apple, respectively. In both graphs, it can be observed that the highest weight losses were generated by the interaction of the conditions of higher concentration and temperature (60°Brix and 45°C). While the lowest losses had a different behaviour, as for melon they were obtained when interacting with the lowest concentration and high temperatures (45°Brix and 45°C). On the other hand, for apple, the use of the lowest concentrations and temperature generated the lowest weight losses (45°Brix and 35 °C).
![]() |
Figure 9: Concentration-temperature interaction on WR during melon osmodehydration |
Regarding water loss (WL), it had a similar behaviour to weight loss, since the greatest losses were generated when using the highest temperature and concentrations (See Figure 10), reaching a water reduction in melon of up to 75.979% and 53.234% in apple for the same conditions (See Table 2). These results may contrast with what has been mentioned by authors who have investigated dehydration processes in various fruits,5,45,46 who agree in pointing out that temperature and concentration have significant effects for weight and water loss.
![]() |
Figure 10: Concentration-temperature interaction on WL during melon osmodehydration |
Table 2: Percentage of water loss during osmotic dehydration of melon and apples
Factor A: Concentration |
Factor B: Temperature |
||
35 °C |
45 °C |
||
Melon |
45 °Brix |
61.220 ± 0.618a |
61.054 ± 0.258a |
60 °Brix |
69.048 ± 0.638b |
75.979 ± 0.286c |
|
Apple |
45 °Brix |
37.510 ± 0.484a |
38.453 ± 0.469a |
60 °Brix |
45.066 ± 0.177b |
53.234 ± 1.026c |
However, Beeu Martinez et al.47 in their melon osmodehydration standard tests, observed that water and weight losses increased when using high concentrations in the solutions (60 and 50 °Brix), while the lowest losses were observed when using 40 ° Brix. Li & Ramaswamy 48, observed the same behaviour in apple cylinders, where the high temperature-high concentration condition (60 ° C and 60 °Brix) gave the highest reduction in weight and water, while the low temperature-low concentration condition (40 C and 40 Brix) gave the lowest reduction among them.
Solid Gain (SG).
Regarding the gain, in solids differences were found in the results obtained for both fruits. While in apples an increase in solids was observed when high concentrations and higher temperatures were used, in melon the opposite behaviour occurred, since the greatest gains were observed in treatments carried out under the lowest concentration studied, 45 ° Brix (see Figure 11).
![]() |
Figure 11: Concentration-temperature interaction on SG during melon osmodehydration |
The ANOVA for the effect of solids gain in apple determined the existence of significant differences with respect to concentration and interaction of factors with p-value <0.05, while for temperature a p-value >0.05 was obtained, denoting that temperature has no significant effect on solids gain, similar values demonstrated by Li & Ramaswamy 48 for solids gain in apple cylinders. The results of the solid tests showed that the gain in the apple was increased when using high osmotic concentrations (60°Brix) with values of 5.532% and 6.725%, differentiating these treatments by the temperature used, being 35°C for the lowest gain and 45°C for the highest (See Table 3). Similar behaviour was found by Salehi 49, who reported an increase in solids in osmotically dehydrated apples with an increase from 5.1% to 9.2% when increasing the concentration of the osmotic solution from 30 °Brix to 50 °Brix.
On the other hand, with respect to the gain of solids in the osmotic dehydration of melon, significance was also found in the effect of concentration and the interaction concentration* temperature with p <0.05, while temperature was not significant (p >0.05). The results show a contrary behaviour to apple, since the highest solid gains (4.153%) were presented when using lower syrup concentrations and higher temperature (45°Brix- 45°C), while the lowest gain of 0.202% was obtained at a concentration of 60 ° Brix and 45°C temperature (See Table 3); a similar result was found by Beeu Martinez et al. 47 who reported that in osmodehydrated melon slices at atmospheric pressure, SG increased when decreasing the concentration and increasing the temperature of the osmotic solution. In osmodehydrated papaya, similarities were observed with respect to solids gain, in this case, this behaviour is due to the fact that in general, little solids gain is obtained when the outflow of water from the feed is faster and more significant, which will depend on the nature of the feed tissue.50
Table 3. Percentage of solids gain during osmotic dehydration of melon and apples
Factor A: Concentration |
Factor B: Temperature |
||
35 °C |
45 °C |
||
Melon |
45 °Brix |
2.375 ± 0.205b |
4.153 ± 0.168c |
60 °Brix |
2.210 ± 0.269b |
0.202 ± 0.098a |
|
Apple |
45 °Brix |
3.324± 0.456b |
2.509 ± 0.160a |
60 °Brix |
5.532 ± 0.240c |
6.725 ± 0.267d |
From the results obtained from the process, it can be deduced that osmotic dehydration is a partial dehydration method. Therefore, in order for the product to remain stable over time, authors such as Ahmed et al. 5 point out that an additional preservation treatment must be carried out, either drying by hot air, freezing, or other processes. One of the methods most commonly used after osmodehydration is conventional hot air drying, since it has been demonstrated that dry products can be obtained with lower nutrient losses, because the food has already lost asignificant percentage of water during osmodehydration, so that the time of exposure to high drying temperatures is usually considerably reduced51–53.
Likewise, the use of sucrose as an osmotic agent has been shown to be quite efficient with respect to weight loss, water and solids gain54. On the other hand, this agent also allows the inhibition of enzymes such as polyphenol oxidase, thus avoiding changes in food coloration due to browning and preserving its appearance55.
About the equipment designed
The equipment obtained and the results found during the tests showed satisfactory results with respect to the comparisons made with other authors, this implied a process where only the equipment itself was needed to obtain data from the process, leaving aside the use of additional equipment such as thermometers or refractometers for taking samples, in this way the manual dependence of the process was notably reduced. These benefits obtained from our equipment, coincide with those indicated by Haleem et al.56 who point out that automated processes contribute to process improvement, as they allow time-consuming processes to be operated using fewer resources and decreasing interaction with equipment. On the other hand, Khan et al. 57 highlights that nowadays almost all processes are automated, due to the benefits it provides in terms of productivity and control.
Likewise, the use of IoT during the process provided great benefits during the process, which address the industrial need to reduce human-machine interaction, allowing a more dynamic process by being able to access process data remotely and thus giving the evaluator the possibility of generating decisions in advance of their process. Various authors58–60, agree on the great benefits of IoT in processes, where they point out that the main benefits are not only limited to automating and monitoring processes, but also the main advantage of this technology is predictive maintenance, since through real-time access to process data, decisions can be taken in advance of possible problems. Also, the use of smart sensors such as the Tilt Float, have a vital importance during the implementation of the IoT, since they are implemented for access to the Internet. These sensors have integrated features that differentiate them from other common sensors, such as auto-detection, intelligent calibration, detection and communication capabilities and can be configured remotely, making them indispensable when implementing technologies such as IoT.61
Conclusions
An osmotic dehydration unit with temperature control and recirculation of the osmotic solution was designed. The IoT was implemented using a smart sensor, which allowed obtaining and monitoring temperature and concentration data remotely and in real time, by connecting it to Bluethooth. An Android application allowed the process data to be stored in the cloud for later analysis. Tests of the equipment on apples and melons demonstrated the correct operation of the equipment, with satisfactory results, obtaining water losses of up to 44.007% for apples and 75.259% for melons. This equipment will allow a more consistent evaluation and monitoring during osmodehydration, and can be used to carry out various investigations regarding the osmodehydration not only of fruits, but also of vegetables.
Acknowledgement
We would like to acknowledge and thank to the National Frontier University for funding the construction of the prototype developed for this research.
Conflicts of Interest
The authors declares that there is no conflict of interest regarding the publication of this paper.
Funding Sources
The construction of the equipment was financed by the Universidad Nacional de Frontera – Sullana, Peru. The authors were among the winners of the ” I Contest for the financing of research leading to the Academic Degree of Bachelor or Professional Degree of the Universidad Nacional de Frontera “, according to the Resolution of the Organising Commission N° 385-2021-UNF/CO.
References
- Krokida MK, Maroulis ZB, Saravacos GD. The effect of the method of drying on the colour of dehydrated products. Int J Food Sci Technol. 2001;36(1):53-59. doi:10.1046/j.1365-2621.2001.00426.x
CrossRef - Cunningham FE. Practical Applications of Food Dehydration: A Review. J Food Prot. 1982;45(5):479-484. doi:10.4315/0362-028X-45.5.479
CrossRef - Guiné R. The Drying of Foods and Its Effect on the Physical-Chemical, Sensorial and Nutritional Properties. International Journal of Food Engineering. 2018;4(2):93-100. doi:10.18178/ijfe.4.2.93-100
CrossRef - Shi JX, Le Maguer M, Wang SL, Liptay A. Application of osmotic treatment in tomato processing—effect of skin treatments on mass transfer in osmotic dehydration of tomatoes. Food Research International. 1997;30(9):669-674. doi:10.1016/S0963-9969(98)00031-3
CrossRef - Ahmed I, Qazi IM, Jamal S. Developments in osmotic dehydration technique for the preservation of fruits and vegetables. Innovative Food Science and Emerging Technologies. 2016;34:29-43. doi:10.1016/j.ifset.2016.01.003
CrossRef - Rahaman A, Zeng XA, Kumari A, et al. Influence of ultrasound-assisted osmotic dehydration on texture, bioactive compounds and metabolites analysis of plum. Ultrason Sonochem. 2019;58:104643. doi:10.1016/j.ultsonch.2019.104643
CrossRef - Bekele Y, Ramaswamy H. Going beyond conventional osmotic dehydration for quality advantage and energy savings. Ethiopian Journal of Applied Science and Technology. 2010;1(1):1-15.
- Flores-Mendoza LC, Calle-Berru EM, Sanchez-Chero M. Diseño y la automatización del proceso de deshidratación osmótica: Una revisión sistemática. Revista Ibérica de Sistemas e Tecnologias de Informação. 2022;E51:82-93.
- Khan PW, Byun YC, Park N. IoT-Blockchain Enabled Optimized Provenance System for Food Industry 4.0 Using Advanced Deep Learning. Sensors. 2020;20(10):2990. doi:10.3390/s20102990
CrossRef - Lage OS. Blockchain: aplicaciones en la industria alimentaria. In: XXXIII Jornada Anual de ACTA/CL Sobre Digitalización En El Sector Agroalimentario. Vol 69. Revista de la Asociación de Científicos y Tecnólogos de Alimentos de Castilla y León; 2019:13-17.
- Dadhaneeya H, Nema PK, Arora VK. Internet of Things in food processing and its potential in Industry 4.0 era: A review. Trends Food Sci Technol. 2023;139:104109. doi:10.1016/J.TIFS.
CrossRef - Sharma BB, Gupta G, Vaidya P, Basheer S, Memon FH, Thakur RN. Internet of Things-Based Crop Classification Model Using Deep Learning for Indirect Solar Drying. Wirel Commun Mob Comput. 2022;2022. doi:10.1155/2022/1455216
CrossRef - Popa A, Hnatiuc M, Paun M, et al. An Intelligent IoT-Based Food Quality Monitoring Approach Using Low-Cost Sensors. Symmetry (Basel). 2019;11(3):374. doi:10.3390/SYM11030374
CrossRef - Mishra N, Jain SK, Agrawal N, Jain NK, Wadhawan N, Panwar NL. Development of drying system by using internet of things for food quality monitoring and controlling. Energy Nexus. 2023;11:100219. doi:10.1016/J.NEXUS.2023.100219
CrossRef - Rajesh BN, Nagamani V, Sowmya G. Temperature and Humidity Sensor Based Air Blower Controller For Food Drying. Journal of algebraic statistics . 2022;13(1):869-876.
- Masetti G, Marazzi F, Di Cecilia L, Rovati L. IOT-Based Measurement System for Wine Industry. 2018 Workshop on Metrology for Industry 40 and IoT, MetroInd 40 and IoT. Published online 2018:163-168. doi:10.1109/METROI4.2018.8428308
CrossRef - Canete-Carmona E, Gallego-Martinez JJ, Martin C, Brox M, Luna-Rodriguez JJ, Moreno J. A Low-Cost IoT Device to Monitor in Real-Time Wine Alcoholic Fermentation Evolution through CO2 Emissions. IEEE Sens J. 2020;20(12):6692-6700. doi:10.1109/JSEN.2020.2975284
CrossRef - Smiljkovikj K, Gavrilovska L. SmartWine: Intelligent end-to-end cloud-based monitoring system. Wirel Pers Commun. 2014;78(3):1777-1788. doi:10.1007/S11277-014-1905-X/METRICS
CrossRef - Popov A, Popov D, Mukhim-Zade M, Wegner N. Industrial internet of things in production of cooked smoked sausages. In: IOP Conf. Series: Earth and Environmental Science . ; 2021. doi:10.1088/1755-1315/640/7/072003
CrossRef - Hu T, Zheng M, Zhu L. Research application of the internet of things monitor platform in meat processing industry. In: Park J, Jin H, Liao XZR, eds. Proceedings of the International Conference on Human-Centric Computing 2011 and Embedded and Multimedia Computing 2011. Springer; 2011. doi:https://doi.org/10.1007/978-94-007-2105-0_17
CrossRef - Teixeira S, Arrais R, Dias R, Veiga G. On the development and deployment of an IIoT Infrastructure for the Fish Canning Industry. Procedia Comput Sci. 2023;217:1095-1105. doi:10.1016/J.PROCS.2022.12.308
CrossRef - Mendoza Montoya JJ, Penalva GT, Navarro EA, Zea KH, Rivera Suana JA, Chilo J. IoT Aroma Sensor Module to Determine Beverage Alcohol Grade. Proceedings of the 11th IEEE International Conference on Intelligent Data Acquisition and Advanced Computing Systems: Technology and Applications, IDAACS 2021. 2021;1:43-48. doi:10.1109/IDAACS53288.2021.9661006
CrossRef - Buonocore D, Ciavolino G, Caro D Di, Liguori C. An IoT-based beer fermentation monitoring system. In: 2021 IEEE International Workshop on Metrology for Agriculture and Forestry (MetroAgriFor). ; 2021:263-267. doi:10.1109/METROAGRIFOR52389.2021.9628536
CrossRef - Kodan R, Parmar P, Pathania S. Internet of Things for Food Sector: Status Quo and Projected Potential. Food Reviews International. 2019;36(6):584-600. doi:10.1080/87559129.2019.1657442
CrossRef - Barbosa EJ, Villada DC, Mosquera AS. Diseño y Construcción de un equipo osmodeshidratador para el desarrollo de productos agroalimentarios. Biotecnología en el Sector Agropecuario y Agroindustrial. 2013;11(1):37-48.
- Gallo LA, Hernandez L, Acevedo D. Design and construction of a vacuum osmotic dehydrator applied to tropical fruits. Contemporary Engineering Sciences. 2018;11(12):583-599. doi:10.12988/ces.2018.8248
CrossRef - Deza KE, Sánchez JG. Construcción y Automatización de Un Equipo Para Secado Por Ósmosis y Su Evaluación En Papaya (Carica Papaya). Tesis. Universidad Nacional del Altiplano; 2011.
- Cahuaya S, Cayo AR. Construcción de Un Equipo de Deshidratación Osmótica Con Pulsos de Vacío y Su Evaluación En Láminas de Papaya (Carica Papaya l.). Thesis. Universidad Nacional del Altiplano; 2010.
- Ispir A, Toǧrul IT. Osmotic dehydration of apricot: Kinetics and the effect of process parameters. Chemical Engineering Research and Design. 2009;87(2):166-180. doi:10.1016/j.cherd.2008.07.011
CrossRef - Siucińska K, Konopacka D, Mieszczakowska-Frac M, Połubok A. The effects of ultrasound on quality and nutritional aspects of dried sour cherries during shelf-life. LWT – Food Science and Technology. 2016;68:168-173. doi:10.1016/J.LWT.2015.11.055
CrossRef - Giovanelli G, Brambilla A, Sinelli N. Effects of osmo-air dehydration treatments on chemical, antioxidant and morphological characteristics of blueberries. LWT – Food Science and Technology. 2013;54(2):577-584. doi:10.1016/J.LWT.2013.06.008
CrossRef - Peiró R, Dias VMC, Camacho MM, Martínez-Navarrete N. Micronutrient flow to the osmotic solution during grapefruit osmotic dehydration. J Food Eng. 2006;74(3):299-307. doi:10.1016/J.JFOODENG.2005.03.022
CrossRef - Seguí L, Fito PJ, Fito P. Analysis of structure-property relationships in isolated cells during OD treatments. Effect of initial structure on the cell behaviour. J Food Eng. 2010;99(4):417-423. doi:10.1016/J.JFOODENG.2009.05.019
CrossRef - Heping Li and Hosahalli S Ramaswamy. Osmotic dehydration. Stewart Postharvest Review. 2006;1(4):1-9. doi:10.2212/SPR.2005.4.3
CrossRef - Vázquez HJ, Dacosta O. Fermentación alcohólica: Una opción para la producción de energía renovable a partir de desechos agrícolas. Alcoholic fermentation: An option for renewable energy production from agricultural residues. Ingeniería, investigación y tecnología. 2007;8(4):249-259.
CrossRef - Jullien C, Bénézech T, Carpentier B, Lebret V, Faille C. Identification of surface characteristics relevant to the hygienic status of stainless steel for the food industry. J Food Eng. 2003;56(1):77-87. doi:10.1016/S0260-8774(02)00150-4
CrossRef - Ciurzy A, Kowalska H, Czajkowska K, Lenart A. Osmotic dehydration in production of sustainable and healthy food. Trends Food Sci Technol. 2016;50:186-192. doi:10.1016/j.tifs.2016.01.017
CrossRef - Sweat VE. Experimental values of thermal conductivity of selected fruits and vegetables. J Food Sci. 1974;39(6):1080-1083. doi:10.1111/j.1365-2621.1974.tb07323.x
CrossRef - Sweat VE. Thermal properties of low- and intermediate-moisture food. ASHRAE Trans. 1985;91(2):369-389.
- TiltHydrometer. Tilt Float Hydrometer for Float Pods – Tilt Hydrometer. Published 2022. Accessed May 22, 2022. https://tilthydrometer.com/products/copy-of-dealer-price-float-tank-hydrometer
- Jaywant SA, Singh H, Arif KM. Sensors and Instruments for Brix Measurement: A Review. Sensors 2022, Vol 22, Page 2290. 2022;22(6):2290. doi:10.3390/S22062290
CrossRef - Kakkar L, Gupta D, Saxena S, Tanwar S. IoT Architectures and Its Security: A Review. Lecture Notes in Networks and Systems. 2021;166:87-94. doi:10.1007/978-981-15-9689-6_10/COVER
CrossRef - Del-Valle-Soto C, Valdivia LJ, Velázquez R, Rizo-Dominguez L, López-Pimentel JC. Smart Campus: An Experimental Performance Comparison of Collaborative and Cooperative Schemes for Wireless Sensor Network. Energies . 2019;12(16):3135. doi:10.3390/EN12163135
CrossRef - Lai X, Yang T, Wang Z, Sciences PCA, 2019 undefined. IoT implementation of Kalman filter to improve accuracy of air quality monitoring and prediction. Applied Sciences. 2019;9(9):1831. doi:10.3390/app9091831
CrossRef - Chandra S, Kumari D. Recent Development in Osmotic Dehydration of Fruit and Vegetables: A Review. Crit Rev Food Sci Nutr. 2015;55(4):552-561. doi:10.1080/10408398.2012.664830
CrossRef - Tortoe C. A review of osmodehydration for food industry. African Journal of Food Science. 2010;4(6):303-324.
- Beeu Martinez B, Abud-Archila M, Ruiz-Cabrera M, et al. Pulsed vacuum osmotic dehydration kinetics of melon (Cucumis melo L.) var. cantaloupe p. Article in African Journal of Agricultural Research. 2011;6(15):3588-3596.
- Li H, Ramaswamy H. Osmotic Dehydration of Apple Cylinders: I. Conventional Batch Processing Conditions. Drying Technology. 2007;24(5):619-630. doi:10.1080/07373930600626545
CrossRef - Salehi F, Cheraghi R, Rasouli M. Mass transfer kinetics (soluble solids gain and water loss) of ultrasound-assisted osmotic dehydration of apple slices. Scientific Reports. 2022;12:15392. doi:10.1038/s41598-022-19826-w
CrossRef - El-Aouar ÂA, Azoubel PM, Barbosa JL, Xidieh Murr FE. Influence of the osmotic agent on the osmotic dehydration of papaya (Carica papaya L.). J Food Eng. 2006;75(2):267-274. doi:10.1016/j.jfoodeng.2005.04.016
CrossRef - Dermesonlouoglou E, Chalkia A, Taoukis P. Application of osmotic dehydration to improve the quality of dried goji berry. J Food Eng. 2018;232:36-43. doi:10.1016/j.jfoodeng.2018.03.012
CrossRef - Soares de Mendonça KS, Corrêa JLG, Junqueira JR de J, Cirillo MA, Figueira FV, Carvalho EEN. Influences of convective and vacuum drying on the quality attributes of osmo-dried pequi (Caryocar brasiliense Camb.) slices. Food Chem. 2017;224:212-218. doi:10.1016/J.FOODCHEM.2016.12.051
CrossRef - Guiamba I, Ahrné L, Khan MAM, Svanberg U. Retention of β-carotene and vitamin C in dried mango osmotically pretreated with osmotic solutions containing calcium or ascorbic acid. Food and Bioproducts Processing. 2016;98:320-326. doi:10.1016/j.fbp.2016.02.010
CrossRef - Corrêa JLG, Ernesto DB, de Mendonça KS. Pulsed vacuum osmotic dehydration of tomatoes: Sodium incorporation reduction and kinetics modeling. LWT – Food Science and Technology. 2016;71:17-24. doi:10.1016/J.LWT.2016.01.046
CrossRef - Quiles A, Hernando I, Pérez-Munuera I, Larrea V, Llorca E, Lluch MÁ. Polyphenoloxidase (PPO) activity and osmotic dehydration in Granny Smith apple. J Sci Food Agric. 2005;85(6):1017-1020. doi:10.1002/JSFA.2062
CrossRef - Haleem A, Javaid M, Singh RP, Rab S, Suman R. Hyperautomation for the enhancement of automation in industries. Sensors International. 2021;2:100124. doi:10.1016/J.SINTL.2021.100124
CrossRef - Khan ZH, Khalid A, Iqbal J. Towards realizing robotic potential in future intelligent food manufacturing systems. Innovative Food Science & Emerging Technologies. 2018;48:11-24. doi:10.1016/J.IFSET.2018.05.011
CrossRef - Yooyativong T, Kamyod C, Kim CG. The Integration of Traditional Rural Solar Dryer with LoRa and IoT Technology. Published online June 8, 2023:434-437. doi:10.1109/ECTIDAMTNCON57770.2023.10139505
CrossRef - Parpala RC, Iacob R. Application of IoT concept on predictive maintenance of industrial equipment. MATEC Web of Conferences. 2017;121. doi:10.1051/matecconf/20171210 MSE 2017 2008
CrossRef - Compare M, Baraldi P, Zio E. Challenges to IoT-Enabled Predictive Maintenance for Industry 4.0. IEEE Internet Things J. 2020;7(5):4585-4597. doi:10.1109/JIOT.2019.2957029
CrossRef - Gupta VP. Smart Sensors and Industrial IoT (IIoT): A Driver of the Growth of Industry 4.0. Internet of Things. Published online 2021:37-49. doi:10.1007/978-3-030-52624-5_3/COVER
CrossRef

This work is licensed under a Creative Commons Attribution 4.0 International License.